According to the introduction, the main utilization method of methane at present is direct combustion. While converting methane into high value-added liquid oxidation products can effectively utilize fossil resources while reducing greenhouse gas emissions.
Therefore, people have always hoped to utilize the abundant methane resources and meet the demand for high value-added oxygen-containing products.
However, selectively catalytic oxidation of methane to produce oxygen-containing compounds is a global challenge and is known as the "Holy Grail" in the field of chemistry. At present, the main products of methane are C1 oxygen-containing compounds.
Ethanol is an important raw material in the chemical, pharmaceutical, and agricultural fields. Traditionally, ethanol is mainly prepared by fermentation of grains, direct hydration of ethylene, and chemical-biological combined operations.
However, the raw materials used in these methods are very susceptible to the supply of climate fluctuations and land resources.Producing ethanol from the abundant methane resources can reduce the dependence on land resources and climate conditions, enhance the stability and resilience of energy supply, and is also a key pathway to achieve efficient utilization of resources such as natural gas and diversification of ethanol raw materials.
Advertisement
At room temperature and atmospheric pressure, the photocatalytic liquid-phase oxidation of methane to ethanol is highly attractive, but it also faces greater challenges.
The reason is: this requires a balance between C-C coupling and hydroxylation. And this is extremely difficult to achieve simultaneously in the high-temperature and high-pressure environment rich in reactive oxygen free radicals commonly used.
Previously, academic research on photocatalytic reduction of gas molecules such as CO2 and O2 has shown:
Introducing a self-supported floating membrane at the gas-liquid interface, by constructing a gas-liquid-solid three-phase interface, can optimize the transmission and dissolution of the gas.This allows for the enhancement of gas transfer to the catalyst by shortening the gas diffusion path, thereby increasing the reaction rate.
This greatly inspired Professor Wang Lingzhi and Professor Zhang Jinlong from East China University of Science and Technology, and they began to consider the limitations of the traditional three-phase interface used for methane oxidation.
In methane oxidation, water plays a key role in promoting the detachment of oxidation products from the solid surface, promoting the generation of reactive oxygen free radicals, and suppressing the occurrence of over-oxidation reactions.
Therefore, in addition to the mass transfer promotion function of the traditional three-phase interface, when designing a three-phase interface for methane oxidation, the impact of water on methane over-oxidation should also be fully considered.
It is also necessary to consider how to controllably generate reactive oxygen species free radicals within the three-phase interface area, and to consider the complex interactions between these processes.It is understood that in their preliminary work, the team had utilized the dynamic contraction and rebound behavior of hydrogels to facilitate the reversible valence transformation of metal ions in Fenton-like reactions.
Furthermore, they combined this hydrogel-like Fenton system with carbon paper to prepare a triphase interface hydrogel catalyst, achieving good methane oxidation activity.
The preparation of the carbon paper hydrogel catalyst is relatively complex, involving interference from organic reagents in product detection, as well as the energy consumption and operational complexity that may be brought by external strong oxidants.
Therefore, they shifted their research focus to a simpler and more efficient method: instead of any hydrophobic modification, they utilized the hydrophilicity and lightweight, porous nature of the hydrogel material itself to prepare a floating triphase interface film, and used photocatalytic water oxidation to generate hydrogen peroxide for the oxidation of methane in situ.
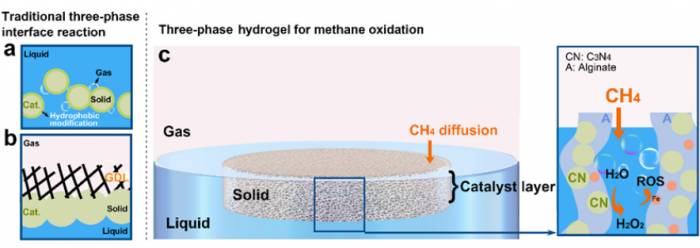
The optimization of the hydrogel film preparation method is an important phase in this research.During this period, they found that when preparing sodium alginate hydrogels through the external gelation method, the rapid gelation speed made it difficult to prepare a uniform hydrogel film.
However, after freeze-drying, the research team observed through scanning electron microscopy that the surface of the aerogel had a porous structure.
They realized that this porous structure could promote the diffusion and mass transfer of gas molecules, thereby solving the problem of methane oxidation in the liquid-phase free radical system.
Subsequently, they further used the method of metal ion cross-linking to prepare it into a hydrogel film. This method can not only complete the gelation process but also maintain the pore structure at the same time.
What is particularly ingenious is that metal ions also have multiple functions, which can act as cross-linking agents, and at the same time, they can also participate in the Fenton reaction, decomposing the in-situ produced hydrogen peroxide into active oxygen species free radicals.In the embedding of photocatalysts, initially they attempted catalysts suitable for the oxygen reduction reaction pathway, and those suitable for the water-oxygen reduction reaction pathway.
After numerous experiments and comparisons, they reached the following conclusions:
When a catalyst that produces hydrogen peroxide through the oxygen reduction reaction pathway is embedded, although it can promote the diffusion of methane and oxygen, it also increases the variety of active species in the system, leading to uncontrollability and complexity in the oxidation products of methane.
Considering that this topic mainly focuses on the design of the hydrogel three-phase interface, a simpler free radical system is needed to elucidate the role and ingenuity of the three-phase interface construction.
Ultimately, they chose photocatalysts suitable for the water oxidation reaction to produce hydrogen peroxide, which, while simplifying the system, can also achieve efficient methane conversion activity and selectivity.At the same time, it also highlights the importance of the design of the triphase interface of the hydrogel in the regulation and optimization of the reaction.
The research group also carried out the optimization of experimental conditions, contamination testing and activity testing of the hydrogel-based catalyst, ensuring the efficiency of the methane oxidation reaction.
They found that the hydrogel triphase interface indeed has high efficiency for methane oxidation at room temperature and atmospheric pressure, and then they began to study and verify the mechanism.
Using electron paramagnetic resonance technology, they found that the concentration of methyl radicals and hydroxyl radicals in the system is matched.
Subsequently, the team conducted more control experiments to verify this mechanism, thereby ensuring the reliability of the research results.By monitoring and analyzing the evolution of the concentrations of various products in the system, the reaction pathway for methane to produce ethanol was further determined, and a deeper understanding of the overall reaction mechanism was enhanced.
In fact, during the research period, they tried many systems. Since methane needs to be activated first, it is basically under high pressure, oxygen-enriched, and even hydrogen peroxide-enriched conditions to explore catalysts that can balance efficiency and selectivity.
The ethanol-selective system was confirmed in the first half of 2023, but compared with other systems they have developed and the liquid-phase oxidation photocatalytic systems of methane reported in recent years, the activity of the aforementioned system is still relatively low.
Therefore, they temporarily put it aside and continued to focus on other catalytic systems to achieve higher conversion rates and selectivity.
The continuous failures during this period also deepened their understanding of the oxygen functionalization of methane.By the end of 2023, Dr. He Chun, who participated in this study, was approaching graduation. Due to the difficulty in advancing in the selective control, she, along with Wang Lingzhi and Zhang Jinlong, proposed to first organize part of the work and publish a paper.
During the process of organizing and discussing, they realized that there was a bias in the original thinking, that is, they overlooked that the systems with relatively high selectivity were basically limited to C1 oxygen-containing products.
In other words: there are very few systems that can take into account both C-C coupling and hydroxylation.
This prompted them to start comparing the differences between the floating hydrogel system and the high-pressure oxygenation system. After repeated discussions and verifications, they had a more unique and in-depth understanding of the selective control mechanism of methane.
Wang Lingzhi said: "We feel that we may have glimpsed a different key point in the selective control of methane. After doing this for such a long time, we feel a little excited and satisfied."During the winter vacation of 2023, and even the days around the Spring Festival of 2024, they have been maintaining frequent contact and discussions.
Despite the use of a porous lightweight floating film in this work, the design concept and preparation process are both very simple, yet it is an effective model to verify whether they understand the key control mechanism.
In other words, the matching of the concentration of carbon-centered radicals and reactive oxygen radicals proposed this time is the key to balancing C-C coupling and oxygen functionalization.
In general, they use a relay water oxidation reaction and a Fenton reaction to control the generation rate of hydroxyl radicals, coordinating it with methane oxidation.
This strategy fully utilizes the functional integration and ease of preparation of hydrogel materials, especially by using the characteristics of hydrogels to construct a three-phase interface.It is reported that through this technology, the efficient utilization of methane resources can be achieved, reducing the emission of greenhouse gases, which helps to address climate change and reduce environmental pollution.
This can transform methane into high value-added oxygen-containing products and further be used in synthetic reactions in the chemical industry.
Among them, hydrogels are beneficial for the assembly of different functional modules and the functional synergy between active components.
In natural oxidases and reductases, the dynamic spatial coordination between the active site and the protein scaffold plays a key role in promoting the transfer of protons/electrons from the enzyme to the substrate.
Therefore, this achievement provides a lot of inspiration for the design of artificial redox catalysts for inorganic-organic composite materials.For instance, elastic structures can assist in achieving synergistic catalysis between metal active centers and surrounding organic co-catalytic components.
Ultimately, the related paper was published in JACS with the title "Photocatalytic Conversion of Methane to Ethanol at a Three-Phase Interface with Concentration-Matched Hydroxyl and Methyl Radicals" [1].
He Chun is the first author, and Wang Lingzhi and Zhang Jinlong serve as co-corresponding authors.
Through this work, they also realized that in the process of exploring and optimizing the catalytic system, facing a pile of failed examples, a few successful ones, and a large amount of data, it is important to be patient and meticulous in analysis and comparison. Understanding the essence of differences is often more important than just focusing on conducting experiments.
In terms of catalysis, low-efficiency systems are often easily abandoned by people. However, low efficiency also varies in different situations.Some systems have low conversion efficiency for the target product because the reaction is too fast and uncontrollable, thus necessitating research in conjunction with in-situ characterization techniques.
However, the sensitivity of the infrared spectroscopy commonly used at present is not sufficient, making it difficult to capture many trace transient information, which increases the difficulty in understanding the reaction mechanism.
On the other hand, low efficiency may also be due to slow kinetics caused by poor mass transfer. Such systems can serve as models for understanding key steps, helping to guide how to further balance efficiency and selectivity.
In the next step, the team will continue to explore the application of hydrogel membrane materials in the field of catalysis.
By continuously optimizing the preparation method, they will continue to work on aspects such as surface super-hydrophobicity and gel mechanical strength, making more contributions to environmental protection and clean energy production.