The new generation of base editors we have developed has tremendous potential in the development of high-version industrial chassis strains. The reviewers have indicated that this is an important breakthrough in the field of gene editing technology, said Han Laichuang, a research assistant at Jiangnan University.
Recently, he and his team have used complex structure-guided Cas protein selection, fusion optimization, and other means to significantly increase the editing window of the base editor to a maximum of 41 nucleotides.
This greatly expands the application range of base editors, making it possible to construct cell mutation libraries using base editors, and also makes it possible to create high-performance chassis strains.
In terms of the working principle of the base editor, the research team has also achieved new breakthroughs. They found that by comparing the sequence and protein structure, it is possible to better determine the key functional sites of Cas proteins and modify them.
In addition, they also explored the impact of different fusion methods on the editing window and associated it with the spatial steric effect of Cas proteins and deaminases.In the study, they tested in two important model bacteria, Escherichia coli and Bacillus subtilis.
Advertisement
By introducing a wide range of mutations and screening at the promoter and ribosome binding site of Bacillus subtilis, they obtained a "promoter + ribosome" binding site combination.
Compared with the expression strength of the starting combination, the expression strength of this combination was increased by 68.1 times.
For the key genes of the Escherichia coli Tat secretion system, the research team also carried out in situ modification, and screened a chassis cell, whose secretion ability was increased by 6.49 times year-on-year.
In general, this study aims at the pain points of industrial strain modification, exploring the impact of factors such as Cas protein selection and deaminase fusion methods on the editing window, and demonstrating the important role of biostructure analysis in the development of gene editing systems.There is an urgent need to develop chassis cells with better performance.
Currently, as issues such as energy supply, food security, and environmental pressure become increasingly severe, how to achieve green and sustainable development has become a topic of common concern for humanity.
Under these circumstances, synthetic biology has emerged. It refers to the guided design, modification, and even creation of "artificial life" with unnatural functions under the guidance of engineering ideas, that is, to achieve the engineering of life systems.
Following the "discovery of the DNA double helix structure" and the "Human Genome Project," synthetic biology is considered the third biotechnology revolution for humanity.
As a foundational technology, synthetic biology has had a profound impact on multiple industries, including bio-fermentation, chemical engineering, food, and medicine, and is rapidly expanding into industries such as the environment, agriculture, materials, and energy.Its extensive application and rapid development demonstrate its enormous potential in achieving grand goals such as green manufacturing, carbon neutrality, and big health.
Undoubtedly, synthetic biology will bring profound changes to traditional production and manufacturing methods. Industrial strains are the "soul" of synthetic biological manufacturing. The creation of high-version industrial chassis strains is a hot topic in synthetic biology research.
This is because by optimizing the chassis strains, the efficiency and output of the biomanufacturing process can be further improved, thereby promoting the rapid development of related industries. Among them, efficient gene editing technology plays a key role.
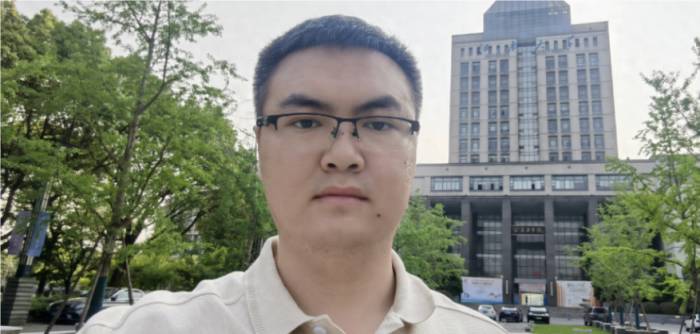
At present, people have developed a variety of gene editing technologies, each with its own characteristics.
Among them, the CRISPR-Cas gene editing technology is a revolutionary gene editing tool that has caused a huge sensation in the biomedical field since its birth in 2012.Compared to traditional gene editing technologies, CRISPR-Cas has the advantages of low cost, easy operation, and high efficiency.
Previously, by utilizing the nucleic acid cutting activity of Cas proteins, people have achieved functions such as gene inactivation, seamless knockout, and exogenous gene recombination.
More importantly, the precise positioning characteristic of CRISPR-Cas brings infinite possibilities to gene editing.
For example, using Cas proteins that have lost their nucleic acid cutting activity and are fused with transcription factors can change the expression intensity of genes, and thus can be used to create a gene expression regulation system.
Furthermore, using Cas proteins that have lost their nucleic acid cutting activity and are fused with proteins with reporter functions can be used as a marking tool, and subsequently used for cell imaging.Unlike gene modification that utilizes the cutting function of the CRISPR-Cas system, when a base deaminase is fused to the Cas protein, with the precise targeting assistance of the Cas protein, the deaminase can act at specific locations in the gene.
This allows the deaminase to directly change the base sequence of the gene through enzymatic catalysis, and people vividly call this system a "base editor."
For this gene editing process, it does not cut the gene, so it will not lead to the destruction of the gene structure. This makes it not only have a high efficiency but also relatively less pressure on the host cells.
In fact, the essence of modifying proteins or cells is to introduce mutations at the gene level to change biological activity.
However, due to the current level of genetic manipulation technology, it is difficult to modify most genes through cloning and in vitro gene engineering operations.Some multi-component systems not only contain larger gene clusters but also have very complex functions. Therefore, in situ modification can only be carried out on the genome.
Base editors are effective tools for in situ gene modification, which can change the gene sequence at specific sites and also construct libraries containing multiple mutations, thereby allowing for phenotypic screening.
However, the editing window problem is one of the main limitations currently faced by base editors.
Previous base editors can usually only edit a range of 5-7 nucleotides, and at most only 2-3 amino acid mutations can occur, which greatly limits the application of base editors.
The reason for carrying out this work is based on the problems encountered by the research group in the long-term study of biocatalysis.From the perspective of enzyme and host compatibility, different enzymes require appropriate hosts for expression, but the effects of expressing different enzymes within the same host can vary greatly.
From the perspective of the expression methods of enzymes, some enzymes are suitable for intracellular expression, while others are suitable for secretion and expression outside the cell.
For this reason, the team has developed a large number of expression systems, such as Escherichia coli, Bacillus subtilis, yeast, filamentous fungi, etc.
For some complex cascade catalytic reactions, it is necessary to strictly control the coordinated expression of enzymes. In addition, some genes can only function within the body and are difficult to clone and modify.
Behind these issues lies a common problem: how to efficiently develop chassis cells with better performance.After conducting some research, they believe that the CRISPR-Cas technology is a better choice to solve the aforementioned problems.
Embrace AI technology actively, providing an important basis for the development of base editors.
Before this, the team had used CRISPR-Cas technology to construct related systems in Escherichia coli, Bacillus subtilis, and Aspergillus niger, achieving gene knockout, gene knock-in, and regulation of gene expression.
On this basis, they decided to focus on the development and application of CRISPR-Cas technology as one of the key points of this research.
As mentioned earlier, although gene editing technology has made certain progress, there are still limitations in dealing with large gene clusters and complex multi-component systems.Therefore, they decided to focus their research on how to expand the editing window of base editors to improve their application scope.
During the experimental design phase, the research team comprehensively considered the impact of various factors such as the selection of Cas proteins and the fusion methods of deaminases on the editing window.
Through comparative analysis and structural prediction, Cas proteins with potential advantages were screened out, and different fusion schemes were tested through design.
At the same time, they also constructed a series of test vectors and chassis strains to verify the performance and application potential of base editors.
By comparing the performance differences of editors under different editing windows, the stability and reliability of constructing a mutation library were evaluated.Utilizing bioinformatics methods, the editing results were predicted and verified, further confirming the accuracy and effectiveness of the editor.
Additionally, they also used the deep learning model-based structural prediction tool RosettaFoldNA to predict and analyze the structure of Cas protein-nucleic acids, which provided important basis for the development of base editors.
Ultimately, the relevant paper was published in Advanced Science (IF 15.1) with the title "A New-Generation Base Editor with an Expanded Editing Window for Microbial Cell Evolution In Vivo Based on CRISPR-Cas12b Engineering."
Jiangnan University Ph.D. student Hao Wenliang is the first author, and Han Laichuang and Professor Zhou Zhe of Jiangnan University are the co-corresponding authors [1].
Subsequently, they will continue to carry out work in three aspects:Firstly, enhance the application capability of in situ transformation technology based on base editors.
At that time, they will carry out the above exploration in typical model bacteria, construct a mutant library for key genes of the biological functional systems of interest, thereby obtaining high-performance chassis cells.
Secondly, conduct research on the applicability of non-model strains.
Although this system has good verification effects in Escherichia coli and Bacillus subtilis, this is far from enough.
There are many industrial strains, many of which are non-model strains. Therefore, they will test this tool in non-model strains, optimize some adaptability issues, and expand the application range of this technology.Thirdly, develop a gene editing system with better performance.
The methods of in situ gene modification are not only numerous but also have their own advantages. Therefore, the research group will still focus on "construction and modification of in situ gene mutant libraries" to develop new gene editing systems.
At the same time, the team is also using AI sequence generation models to design artificial deaminases, optimize the fusion of Cas proteins and deaminases, etc.
It believes that the rapid development of AI will definitely subvert the traditional biological technology research methods, that is, from the previous mechanism-driven (understand first, then design) to data-driven (design first, then understand).