Sir George Malliaras, a Fellow of the Royal Society, a Member of the European Academy of Sciences, and the Prince Philip Professor of Technology at the University of Cambridge, is a leading figure in the field of organic bioelectronics. His related papers have been cited more than 50,000 times, with an h-index as high as 120.
Not long ago, the team's Marie Curie Postdoctoral Fellow, Chaoqun Dong (who graduated with a PhD from the Swiss Federal Institute of Technology in Lausanne), and his colleagues developed a new type of flexible neural interface technology, which led to the creation of a "bio-implantable electrochemical actuator microelectrode" (i.e., a flexible neural sleeve).
This device is based on a 4-micron polymer film, with the entire device thickness around 10 microns, thinner than household plastic wrap, and almost imperceptible when held in hand.
By applying an extremely small voltage, this device, as thin as silk, can be precisely and flexibly stably wrapped around the nerves, thereby achieving a minimally invasive peripheral nerve interface.
This can achieve a safer and more effective neural interface, providing a new solution for minimally invasive diagnosis and treatment of neurological diseases and prosthetic control.Specifically, this technology can be applied to a range of neurological disorders, such as the diagnosis and treatment of epilepsy and chronic pain.
Advertisement
It can also be used for prosthetic control, with the potential to significantly reduce the invasiveness and risk of surgery.
Not only that, but for monitoring neural activity during surgery, this technology can also provide simple and easy-to-operate strategies to increase the success rate of operations.
At the same time, this technology uses a thin-film electronic sleeve design, which can roll itself into a small bundle, thereby being placed inside a needle tip.
In this way, it can be percutaneously implanted during minimally invasive surgery, then controlled by clinical doctors to unfold in situ, and then roll up again.Through this, devices can be placed in areas with highly complex nerve distribution that are difficult to reach by traditional surgery, targeting auditory, visual, and pain nerves for control, thereby avoiding the traditional highly invasive surgical approach of placing implants inside the brain.
Neurological diseases number more than 600, troubling humans for many years.
According to the World Health Organization, neurological diseases have affected over one-third of the global population and are one of the main causes of illness and disability.
There are more than 600 types of neurological diseases, including epilepsy, stroke, migraine, Alzheimer's disease, Parkinson's disease, etc.
This has brought immense pain to many individuals and families, not only depriving the workforce but also causing many losses to society and the country.Looking globally, the monitoring, diagnosis, and treatment of these neurological diseases have always been a thorny problem.
It is understood that peripheral neuropathy - which refers to damage to the nerves outside the brain and spinal cord - usually leads to pain, numbness, and also affects bodily functions such as digestion and urination.
For patients with peripheral nerve diseases, their pain is like a burning or stabbing pain like a knife cut, and this disease, as well as the impact on bodily functions, severely reduces the quality of life of the patients.
Surprisingly, implantable bioelectronic devices can be used to block or stimulate nerve signals.
As a precise treatment plan, these devices not only bring new hope to patients, but also can achieve prosthetic control.For instance, intractable pain can be alleviated by blocking pain signals, or the movement of paralyzed limbs can be restored by sending stimulating electrical signals to the nerves.
However, the electronic devices currently used for peripheral nerve interfaces are not only outdated in design but also have low electrode array resolution, which cannot adapt to nerve fibers of different diameters.
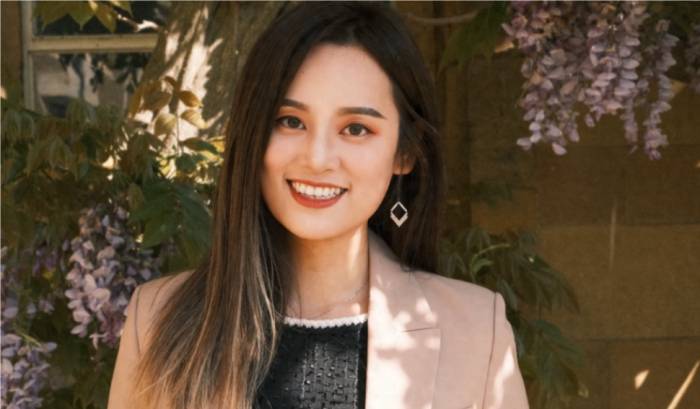
Under such circumstances:
If the contact is too tight, it will exert pressure on the nerve, leading to impaired blood circulation and degeneration of nerve function;
If the contact is loose, it will result in poor electrical contact and may cause electrode displacement.Additionally, these devices require complex surgical procedures during implantation, leading to a higher risk of nerve damage.
So, how can we achieve high-quality neural stimulation and signal recording while reducing nerve damage and surgical risks?
Dong Chaoqun has always been very interested in medical health, especially the study of neurological diseases.
Previously, during her doctoral studies at the Swiss Federal Institute of Technology in Lausanne, she laid a certain foundation in soft materials and electronic devices.
After graduating with her doctorate, she intends to continue her research as a postdoctoral fellow, hoping to focus on exploring neurological diseases.All human activities, such as the function of internal organs, body movements, perceptions, and even emotions, are controlled by the nervous system.
"If I can help people understand these diseases through the development of materials and devices, or improve the success rate of treatment and reduce pain by reducing the invasiveness and side effects of surgery, then I think my efforts are valuable," said Dong Chaoqun.
So, she contacted Professor George Malliaras of the University of Cambridge (who is also Dong Chaoqun's current postdoctoral supervisor) to inquire and discuss the possibility of applying for the Swiss Postdoctoral Fellowship.
Professor George is a leading figure in the field of international organic semiconductors and organic bioelectronics. "And the idea of this work was born in the process of our discussion, combining my previous knowledge and experience in soft actuation, soft material preparation, electrochemistry, and electronic devices," said Dong Chaoqun.
After that, Dong Chaoqun carried out further literature research and preliminary design, and after finding it feasible, she wrote a postdoctoral fellowship application and successfully obtained the funding.In close contact with the sciatic nerve of rats, forming a self-closing neural electronic interface.
In November 2021, she officially came to the University of Cambridge for postdoctoral research. At that time, no one in the laboratory had worked on projects in the direction of electrochemical driving.
To complete this research, Dong Chaoqun took the lead in material preparation and the construction of the testing device.
And although she had no experience in micro-nano processing, with the help of other colleagues in the research group, Dong Chaoqun quickly learned to prepare high-precision thin-film electronic devices.After continuous testing and optimization, the expected effect was ultimately achieved: even without external voltage, the device can form effective contact with fine nerves.
In summary, the team, combining the latest advancements in soft robotics and flexible microelectronics, has proposed this new type of flexible neural interface technology.
In the research, the research group effectively combined conductive polymer soft actuators with low-impedance microelectrodes.
Thanks to its ultra-thin characteristics, the above combination can well ensure the shape adaptability of the device, thereby achieving flexible attachment with objects of highly complex shapes.
By applying an extremely low voltage of less than one volt, the flexible neural sleeve can gently and actively grasp or wrap around fine and fragile peripheral nerves without causing damage to them.The experiment shows that in a living rat model, this device can closely contact the rat's sciatic nerve and can form a reliable self-closing neural electronic interface.
Different from the traditional sleeve design, this new technology can ensure the tightness and stability of the interface without the need for surgical suture threads or glue.
And the seamless combination of this soft electrochemical actuator and the neural interface microelectrode array can provide a new method for realizing minimally invasive intraoperative neural activity monitoring and high-quality bioelectronic interfaces.
"Seeing hallucinations after watching for too long?"During the research process, two interesting incidents also accompanied the work.
At the beginning of the research, Dong Chaoqun made a simple electrochemically driven thin film. When voltage was applied to the film, she expected to see deformation. However, after numerous experiments, the film did not show any deformation.
After several optimizations and improvements, the film was finally able to lift slightly, but Dong Chaoqun was initially a bit skeptical, thinking that she might have been hallucinating from observing for too long.
Therefore, she further optimized the preparation process, and soon achieved a qualitative breakthrough from zero to one: the thin film device could not only lift at an extremely low voltage, but it could also roll up like a blanket into several circles.
At this point, Dong Chaoqun recorded a video of the film's movement with her mobile phone and rushed to Professor George's office to share this progress for the first time."He seemed even more excited than I was, and he even asked me to send him the video so he could share it with his friends and collaborators," said Dong Chaoqun.
During her research period, which coincided with the Christmas season, Dong Chaoqun accidentally noticed the Christmas decorations on the streetlights. This inspired her: it would be great if neural interface devices could also be wound around nerve fibers like this.
If it could be achieved, compared to traditional cuffs, this method could act on a longer segment of the nerve, and it could also ensure close contact at places where the nerve diameter changes.
So, she began to think about how to achieve this deformation. Initially, she thought that asymmetry needed to be introduced in the design.
After reviewing some literature, Dong Chaoqun was even more convinced that asymmetric design was the key to forming spiral deformation.Subsequently, she started with the simplest designs and methods, turning the continuous electrochemically driven parts into slanted strips.
During the device preparation, Dong Chaoqun used high-precision methods, making it easy to create such distributed strips.
"During the test, it was indeed easy to deform in a spiral shape, just as I expected!" she said.
Subsequently, she organized the results of this study into a paper. Finally, the related paper was published in Nature Materials (IF 41.2) with the title "Electrochemically actuated microelectrodes for minimally invasive peripheral nerve interfaces."
Dong Chaoqun is the first author, and Professor George Malliaras of the University of Cambridge served as the corresponding author [1].Figure | Related paper (Source: Nature Materials)
Next, the research team will further explore the peripheral nerves of mammals, such as pigs, and will study the effects of the research device in long-term neural signal collection and stimulation.
They also hope to have the opportunity to test on humans to achieve the transformation of related results.
In addition, the team will also use AI to extract and analyze the signals collected by the device, in order to expand the applicable range of this type of device.
And by using AI to control the stimulation intensity of the device, to achieve more precise and real-time control of the software device actions.At the same time, they will continue to develop new soft robot materials and devices, applying this principle to other neural stimulation or signal acquisition devices.
Thus, it can be implanted into the brain, spinal cord central nervous system, thereby providing a low-risk minimally invasive surgical method for the treatment of neurological diseases.