For many years, Professor Jiang Ying of Peking University and the team led by Academician Wang Enge have been researching the structure of water. The Weiming Lake on the Peking University campus has also become a source of inspiration for him, giving birth to a Nature paper.
One winter day, as he was walking by Weiming Lake, he noticed that when the lake surface froze, the texture of the ice surface was both like water and like ice.
Earlier, in 2008, the team led by Academician Wang Enge had theoretically revealed the close relationship between the stability of the ice surface structure and the arrangement of hydrogen atoms on the surface.
At that time, Jiang Ying's team was already able to distinguish the hydrogen order of the two-dimensional ice surface in experiments. Therefore, this observation at Weiming Lake inspired his strong interest in exploring the actual distribution of hydrogen order on the ice surface.
After returning to the team for discussion, he and his team decided to use scanning probe technology, which is sensitive to hydrogen nuclei, to explore the structure of the ice surface and the pre-melting phenomenon, especially the characteristics at the atomic scale.Through meticulously designed experiments, they successfully "cultivated" the most common hexagonal ice found in nature on a metallic substrate. The thickness of this ice layer reaches several hundred nanometers and is completely insulating.
Advertisement
Later, they further overcame the challenge of in-situ tip modification on the surface of insulators, developing a universal carbon monoxide tip preparation technology.
This technology is applicable to non-intrusive atomic-level imaging of various surfaces, paving the way for observing completely insulating ice surfaces using a qPlus-type scanning probe microscope.
By utilizing qPlus-type low-temperature atomic force microscopy technology, the team solved key issues in the structure and pre-melting phenomena of the ice surface, advancing the understanding of ice's atmospheric reactions and interstellar processes.
Not only does this help resolve long-standing scientific debates, but it also provides new perspectives for exploring the origins of life and the sources of matter.In general, for the study of ice science, this achievement opens a new perspective from the atomic scale and is expected to have a profound impact on fields such as materials science, tribology, biology, atmospheric science, and interstellar chemistry.
It overturns the original understanding of the ice surface: that is, the ice surface is not as perfect as previously thought, but merely a simple cross-section of the ice Ih phase.
In detail, the ice surface exhibits two stacking methods, Ih and Ic, and they are arranged in an alternating manner.
Under suitable crystallization conditions, the domains of these two stacking methods can become equally large, thus forming a new ordered superstructure with the lowest formation energy, which the research group calls "imperfect perfection."
This alternating arrangement also leads to the ice surface being covered with nano-scale "cracks," which are composed of boundary defects.This phenomenon allows ice to undergo premelting at extremely low temperatures (120K), far below the previously widely accepted temperature of over 200K, and comparable to some of the extreme low-temperature environments known on Earth.
This discovery indicates that in the natural environment, most ice surfaces are already in a disordered state of premelting or nearly in a liquid state.
Therefore, studying the surface defects and local metastable structures generated during the premelting process is of great significance for a deeper understanding of the physical and chemical behavior of ice.
For long-standing issues in academia such as the lubricating properties of ice and the excellent catalytic activity of ice at low temperatures, this achievement may provide some answers.
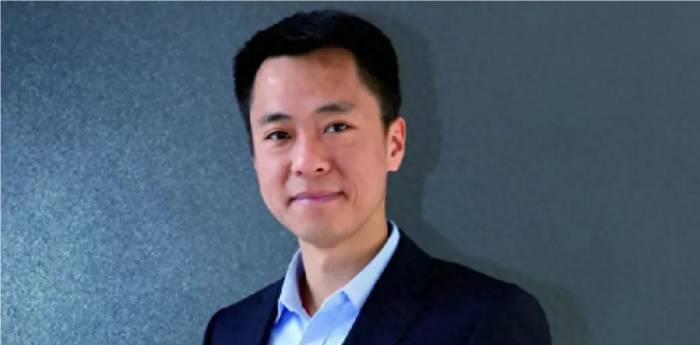
For the first time, atomic-resolution images of hydrogen bonds on the surface of an insulator have been obtained.Additionally, this is also the first time that the academic community has obtained atomic-resolution images of hydrogen bonds on the surface of an insulator.
In the field of nanoscience, scanning tunneling microscopy (STM) and atomic force microscopy (AFM) are two major tools frequently used by researchers when studying surfaces.
Since its invention, scanning tunneling microscopy has rapidly solved the long-standing problem of the 7x7 reconstruction structure on the silicon surface that had been troubling the academic community, and the relevant scholars were awarded the Nobel Prize in 1986.
However, due to the limitation of tunneling electrons, scanning tunneling microscopy can only detect conductive materials.
To break through this limitation, people have utilized the interatomic interaction forces, hoping to apply this technology to insulating materials. In 2016, relevant scholars received the Kavli Nanoscience Prize for their achievements.However, due to the complexity of interatomic forces, the resolution of atomic force microscopy (AFM) is generally not as good as that of scanning tunneling microscopy (STM), and it is difficult to obtain atomic resolution images of the surface.
The qPlus type force sensor and tip decoration technology have since broken this status quo, allowing the resolution of atomic force microscopy to surpass that of scanning tunneling microscopy, and presenting the ability to resolve at the level of chemical bonds.
However, this tip decoration technology can only be performed on metal surfaces. The non-in situ tip decoration technology developed by the team can transfer the decorated single-atom tip to any surface in a non-destructive and repeatable manner, breaking through the limitation that the tip can only be processed on metal surfaces.
This means that atomic-level surface imaging of many difficult-to-process tip surfaces, such as oxides, salts, perovskites, and other functional materials, will become possible, thereby bringing significant impact to the field of material surface science.Developed the domestically produced prototype of the qPlus type scanning microscope and achieved collaboration between universities and enterprises.
In fact, at the beginning of the research, the team originally planned to use foreign equipment for exploration, but the quality of the data obtained was always unsatisfactory.
To further improve the quality of research, they decided to develop domestic equipment. In 2022, the team independently developed a domestically produced prototype of the qPlus type scanning microscope and transferred the technical achievements to Beijing Huairou Zhongke Aikemi Company.
This not only achieved collaboration between universities and enterprises, but also promoted the process of domestication of the qPlus type scanning microscope as a whole.
"The microscope has reached the international leading level in performance, especially in reducing the amplitude noise of the atomic force sensor and improving the quality factor," said Tian Ye, a specially appointed researcher of Jiang Ying's team.Based on the aforementioned domestic research equipment, they were able to perform atomic-level resolution imaging on the most common hexagonal ice surface in nature for the first time, achieving a long-sought goal in academia.
"This not only reflects the progress of domestic research equipment but also highlights the ability of Chinese researchers to innovate independently," the team said.
This is not only China's first qPlus-type scanning probe microscopy system with independent intellectual property rights, but they have also made many innovations and improvements in the design.
For example, by optimizing the design of the shielding cover, the overall thermal loss of the equipment was reduced, allowing the lowest temperature of the scanning head to be lowered from 5.6K to 4.4K, which is already very close to the temperature of liquid helium (4.2K).
Dr. Hong Jiani of Jiang Ying's team said: "Don't look down on this 1K temperature increase, it can ensure that during sample replacement, the temperature rises less and drops faster, greatly improving the success rate of non-in situ needle tip replacement technology, from the previous 60% to 90%."It can be said that the improvement of the signal-to-noise ratio (SNR) is particularly important for analyzing complex hydrogen bond network systems. The research group has cumulatively designed five versions of force sensor coupling methods and manually crafted the qPlus force sensors.
At the same time, they also optimized the circuit structure and assembly method of the liquid helium operational amplifier. They improved the connection of the signal lines and the size of the capacitors, ultimately increasing the SNR by two orders of magnitude, allowing it to approach a quality factor of 100,000, thereby obtaining a clear image of the surface of bulk ice.
When the research group obtained the high-resolution atomic imaging of the ice surface for the first time, they were very careful to transfer a single molecule of carbon monoxide to the ice surface for imaging.
After multiple failures and optimizations, they finally succeeded in obtaining atomic resolution images of the ice surface.
However, the excitement was quickly replaced by confusion. The observed ice surface was quite different from the previous understanding of the academic community. Moreover, no matter how the samples were grown, the ice surface always appeared to be in a disordered state.Initially, they suspected that this was due to the temperature control during the sample growth process not being precise enough.
Therefore, they optimized the control system for growing the samples, ensuring that every parameter was as accurate as possible, and controlled the temperature fluctuations to within 0.03 K.
However, even with these measures, they still could not achieve a structure that only had the Ih order.
After a long period of exploration and repeated experiments, the research team finally discovered that the ice surface could indeed form a long-range ordered superstructure with alternating Ih and Ic arrangements.
At this point, they suddenly realized that the previous samples were disordered because they had undergone pre-melting during the growth process. With this discovery, the research essentially came to an end.Ultimately, the related paper was published in Nature with the title "Imaging surface structure and premelting of ice Ih with atomic resolution" [1].
Dr. Jiani Hong from Peking University (now a postdoctoral fellow at Peking University), Dr. Tian Ye (now a distinguished research fellow at Peking University), PhD student Tiancheng Liang, and PhD student Xinmeng Liu are the co-first authors.
Professor Ying Jiang, Professor Limei Xu, Distinguished Research Fellow Tian Ye, and Academician Enge Wang from Peking University are the co-corresponding authors.
One of the reviewers stated: "This is one of the most impressive and complete papers I have read in many years."
In the review comments, the innovative application of the qPlus atomic force microscope in the study of ice surfaces was affirmed, as well as the unprecedented resolution it brought.The article to be translated into English is:
Investigating the microstructure of the ice surface and the pre-melting process has broad significance and is likely to have a profound impact on various fields such as atmospheric science and materials science.
Exploring the "No Man's Land" of Water Molecules
Of course, understanding the mysteries of the ice surface structure is just the beginning of this series of explorations.
Next, they will delve into the rapid dynamics of water molecules transforming into a solid state under supercooled conditions, a process known as the "No Man's Land" due to its difficulty in being captured and studied.
To this end, they plan to combine femtosecond infrared laser technology with a scanning probe microscope system, achieving precise control of the bulk ice surface through transient heating and ultra-rapid cooling.
The significance of this research is highlighted by the potential widespread impact it could have on fields such as atmospheric science and materials science. By understanding the microstructure of ice surfaces and the pre-melting process, new insights and applications can be developed.
The exploration of the "No Man's Land" of water molecules represents a challenging frontier in the study of ice. The rapid dynamics of water molecules transitioning from a supercooled state to a solid state are difficult to capture and study, making this an area of intense research interest.
To advance this research, the team plans to employ a combination of cutting-edge technologies. They will use femtosecond infrared laser technology in conjunction with a scanning probe microscope system. This innovative approach will enable precise control of the ice surface by applying transient heating and ultra-rapid cooling techniques.
By leveraging these advanced methods, the researchers aim to gain a deeper understanding of the complex processes that occur at the molecular level during the transformation of water molecules into ice. This knowledge could lead to breakthroughs in various scientific disciplines and contribute to the development of new materials and technologies.In an ultra-high vacuum environment, it is expected to achieve a wide range of temperature variations from 5-300K, thereby providing an important technical foundation for revealing the structure of quasi-liquid water, constructing the phase diagram of water, and exploring the "no man's land."
As previously mentioned, as the site for many chemical reactions, the ice surface has profound scientific research value in the fields of atmospheric chemistry, interstellar chemical reactions, and the origin of matter.
Therefore, they plan to combine various spectroscopic techniques with scanning probe microscopy to construct a scanning probe microscopy system that has both chemical resolution and high spatial resolution.
With the help of this combined system, it is expected to conduct in-depth multi-dimensional analysis on the composition of surface chemical elements, the adsorption mechanism of molecules, and the regulation of chemical reactions.
At the same time, although this research group mainly studies physical topics, they have begun to actively embrace artificial intelligence technology.Previously, by combining molecular dynamics simulation with machine learning, the team developed a method capable of predicting the atomic-level structures corresponding to experimental images of water molecular networks.
Further, they utilized transfer learning technology to retrain the neural network, allowing it to demonstrate excellent predictive performance when processing more complex ionic hydrate systems with only two thousand sets of data.
Statistical results show that the algorithm achieved a prediction accuracy of 95% for ions (sodium and potassium ions) and oxygen atoms on simulated data, and the prediction accuracy for hydrogen atoms also reached as high as 85%.
Even without experimental data training, this algorithm can still provide valuable predictions for experimental data of hydrated sodium ions and hydrated potassium ions.
This algorithm not only greatly expands the application of atomic force microscopy in the study of complex interfaces, but also its economical and efficient machine learning process framework is of significant reference value for the analysis of results from other electron microscopy and spectroscopy techniques [2].In the future, they plan to further efficiently integrate artificial intelligence technology with scanning probe systems to achieve automation, standardization, and intelligence in experimental operations and data processing.
It is expected that this will provide a solid experimental foundation for their exploration of the "structure-function relationship" of materials, the complex structure of liquid water, and the interactions between biological macromolecules and water molecules.