"We have achieved the most advanced level of laboratory-scale all-non-precious-metal-based anion exchange membrane electrolysis for hydrogen production to date, and have completely freed ourselves from the traditional iridium/platinum precious metal catalysts," said Sun Licheng, a distinguished professor at Westlake University and an academician of the Chinese Academy of Sciences.
Not long ago, he led his team to propose a stable anion exchange membrane construction strategy, achieving high-performance all-non-precious-metal catalyst anion exchange membrane electrolysis of water (AEM-WE, Anion Exchange Membrane Water Electrolyzers) [1].
Under the working conditions of 2.0V and 80°C, it can achieve a current density of 8A/cm². At the same time, this precious metal-free AEM-WE can maintain long-term operation for more than 2400 hours under gradient constant current test conditions.
At present, the stability of AEM is one of the main obstacles to the large-scale industrialization of AEM-WE. This study fundamentally solves the degradation problem of cationic functional groups in AEM, greatly improving the alkaline stability of AEM.
Advertisement
At the same time, this construction strategy can also be referenced in the development of solid electrolytes for AEM devices such as fuel cells, flow batteries, and metal-air batteries to improve the durability of the devices.Researchers have achieved efficient hydrogen production through electrolysis of water by coupling all-noble-metal anode and cathode catalysts, demonstrating their great potential to replace traditional noble-metal catalysts.
The all-noble-metal-based AEM-WE (Anion Exchange Membrane Water Electrolysis) can greatly reduce the cost of hydrogen production and improve efficiency, showing a promising prospect for commercialization.
It is worth noting that the stability of this new type of AEM under extreme current densities has been preliminarily verified, making it possible for AEM-WE to operate effectively over long periods under high industrial current densities.
For a long time, the AEM field has lacked a representative product similar to the perfluorinated proton exchange membrane (Nafion) in the proton exchange membrane field.
Sun Licheng said: "The poly(aryl-quinuclidinium) AEM we proposed exhibits excellent non-in situ alkaline stability and in situ device stability, and is expected to become a representative AEM product."Completely free from traditional iridium/platinum noble metal catalysts.
This research took a total of two years, and by introducing highly stable AEM, an AEM-WE with both high performance and high stability was achieved.
From the exploration of polymerization conditions to the optimization of device performance, and the discussion of the stability mechanism, the researchers conducted meticulous research.
Researchers started at the molecular level, focusing on the degradation mechanism under cationic alkaline environments. However, initially, attempts to catalyze quinone and aryl polymerization with acid always failed.In a certain discussion, they noticed that the hydrochloric acid inherent in quinoline hydrochloride might be the "culprit" hindering the polymerization. By reducing acidity, they managed to hinder the polymerization reaction.
So, researchers attempted to neutralize quinoline hydrochloride with a base, and after obtaining pure quinoline, they successfully resolved the polymerization issue.
Sun Licheng stated that although small molecule reactions can proceed normally, condensation polymerization is a continuously accumulating reaction process, during which the impact of "impurities" is often more prominent. Therefore, it is essential to eliminate these "impurities" in experiments.
The experimental results showed that the alkaline stability of branched poly(aryl quinoline) is significant. By introducing a rigid cage-like quaternary ammonium salt structure, the bond angle of the β-H was changed, which restricted the degradation of the quaternary ammonium salt, thereby enhancing the stability and overall performance of AEM.
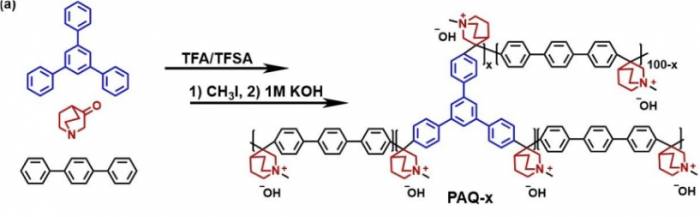
This strategy and the cage-like quaternary ammonium salt structure lay an important foundation for the subsequent development of alkaline-stable AEM, further promoting the advancement of AEM-WE technology.Looking back on the research process, the research team also had a "small episode": Just as this study was basically completed and the device performance was continuously optimized, they found that similar research was published first [2].
This is also a relatively common phenomenon in the field of scientific research. As a result, the researchers conducted a more in-depth study of the degradation mechanism of the AEM, comprehensively improving the research from small molecules to polymers.
Ultimately, this AEM construction strategy achieved the desired performance effect. Under the working conditions of 2.0V and 80°C, it achieved a current density of 8A/cm2, and maintained stable operation for more than 2400 hours under gradient constant current test conditions.
"Frontier scientific research is highly competitive, and the similar research ideas of different research groups can lead to the 'collision' of results at any time. Therefore, there must be a sense of urgency in scientific research, and one should complete their own scientific research projects and topics as soon as possible." Sun Licheng summarized.
The US Department of Energy (DOE, Department of Energy) has set the target requirements for the proton exchange membrane electrolyzer technology in 2025 to reach at least 2A/cm2 under the condition of 1.8V.He stated: "The most exciting moment in the research was when the new strategy we proposed exceeded the DOE's requirements for all non-noble metal-based AEM-WE performance. The team was greatly encouraged and further strengthened our determination to delve deeper into this direction."
Recently, the related paper was published in Angewandte Chemie International Edition[1] with the title "Stable Anion Exchange Membrane Bearing Quinuclidinium for High-performance Water Electrolysis."
Xi Hu University Ph.D. student Yin Liqiang and Assistant Researcher Dr. Ren Rong are the co-first authors, and Professor Sun Licheng serves as the corresponding author.
One of the reviewers believes that the study demonstrates the industrial application potential of AEM-WE technology.
Another reviewer commented: "This study is valuable because it presents a systematic research approach, including achieving high performance of AEM-WE, and demonstrates the potential of quinolinium groups as cationic groups in AEMs, thereby contributing to the development of a new generation of AEMs."It is anticipated that a rapid development phase will be entered within 5 years.
The AEM-WE combines the advantages of proton exchange membrane electrolyzers and alkaline electrolyzers, with the use of non-precious metal catalysts being its greatest cost advantage.
The performance of AEM-WE is synergistically influenced by the catalyst, membrane, assembly process, and other factors. However, the performance of AEM-WE reported in current research is based on precious metal catalysts, which hinders its transition from the laboratory.
Thanks to the development of efficient catalysts and highly stable membranes by this research group, as well as the optimization of assembly processes, a technological breakthrough has been achieved in laboratory-level AEM-WE devices, providing ample assurance for the subsequent scaling up and transformation of this technological achievement.The non-precious metal-based AEM-WE in this study has greatly leveraged the advantages of the device (truly realizing the cost benefits of AEM-WE) and has exhibited excellent performance comparable to that of precious metal catalysts, showing a promising future.
While recognizing the progress in achievements, it is also important to understand that the initial commercialization of AEMs generally suffers from insufficient alkaline stability, and thus there is still some distance to go before actual industrial application.
At present, the industrialization of AEMs is in a rapid development stage, with related technologies and applications continuously maturing and being promoted.
The strategy proposed in this study of introducing rigid cage quaternary ammonium salts to eliminate degradation may become an effective approach. With further technological advancements and increased market demand, the industrialization of this type of AEM is expected to achieve greater breakthroughs and progress.
Currently, there is a demand for highly stable AEMs in fields such as fuel cells, flow batteries, electrodialysis, and metal-air batteries.Sun Licheng pointed out that, relatively speaking, the research on AEM water electrolysis is currently in a leading position and closer to industrialization. Therefore, promoting technology at the right time is beneficial to the rapid development of other fields.
"The anion exchange membrane electrolyzer is a direction that will develop rapidly in the next few years. Currently, there are already some good research results on anion exchange membrane electrolyzers in the academic community, and a large number of AEM-WE products will be launched in a short time. I believe that this field is expected to enter a period of rapid development within 5 years," he said.
Promoting technology from "the shelf" to "the shelf"
Professor Sun Licheng has been associated with "water" for nearly 30 years, long-term engaged in the research of molecular catalysts for water oxidation, and catalyzing water oxidation is the key to preparing green hydrogen.He is one of the "Highly Cited Researchers" globally and has served as a Chair Professor of Molecular Devices at the Royal Institute of Technology in Sweden. In 2013, he was received by the King of Sweden as one of four distinguished scientists; in 2017, he was elected as a member of the Royal Swedish Academy of Engineering Sciences. Additionally, Sun Licheng also received the International Science and Technology Cooperation Award of the People's Republic of China for the year 2018.
In 2012, Sun Licheng's research team achieved a significant breakthrough in the field of artificial photosynthesis: the development of the world-leading water oxidation molecular catalyst Ru-bda, whose catalytic efficiency can be compared with the natural photosynthesis water oxidation center [3].
During his 28 years of work abroad, he has continuously addressed key scientific issues in the field of artificial photosynthesis, accumulating a profound research foundation, mainly including: the design and synthesis of efficient water oxidation catalysts, the mechanism of O-O bond formation, the design of photolytic water splitting hydrogen production devices, photoelectrocatalytic reduction of carbon dioxide/nitrogen, and the design and preparation of new perovskite solar cell hole transport materials.
In March 2020, during the pandemic, Professor Sun Licheng chose to return to his home country to join Westlake University full-time, serving as a Chair Professor of Chemistry in the School of Science and Director of the Center for Artificial Photosynthesis and Solar Fuels, and established the Westlake University Center for Artificial Photosynthesis and Solar Fuels, which now has more than 80 members.
The team focuses on the two major scientific frontiers of solar fuels and solar cells as the main research directions, focusing on major theoretical innovations in basic research and breakthroughs in the bottleneck of application technology.Sun Licheng stated that after returning to China, engaging in research on electrolytic water splitting for hydrogen production was an adjustment in direction that came naturally. Utilizing electrolytic water technology coupled with renewable energy to produce green hydrogen, providing clean energy, promoting energy transformation, and thereby reducing greenhouse gas emissions, is the most promising solution.
Therefore, the core directions of the team include: anion exchange membranes, oxygen evolution reaction (OER), hydrogen evolution reaction (HER) catalysts, and membrane electrode assemblies (MEA).
That is, the 12 English letters "AEM," "OER," "HER," and "MEA." While achieving a series of breakthrough core technologies, the team has also collaborated with many enterprises in research, demonstrating performance superior to market products and obtaining multiple invention patents.
Based on this research, in the next phase of research, Professor Sun Licheng will lead the team to continue focusing on key materials and technologies of AEM-WE, developing all-non-precious-metal anode and cathode catalysts and new stable AEMs, studying specific scientific issues such as key interface mass transfer, device degradation mechanisms, and striving to achieve industrialization of anion exchange membrane electrolyzers in a short period of time.
From "the bookshelf" to "the shelf" is the social responsibility of scientific researchers and has always been the goal of this research group. Accelerating the industrialization of AEM-WE requires comprehensive cooperation between researchers and enterprises in different fields to evaluate and optimize engineering scale-up, material matching, assembly processes, and reliability."We have always upheld a proactive and open attitude, looking forward to working with the industry to promote the popularization and application expansion of AEM-WE, and achieve a win-win cooperation," said Sun Licheng.